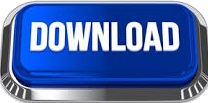
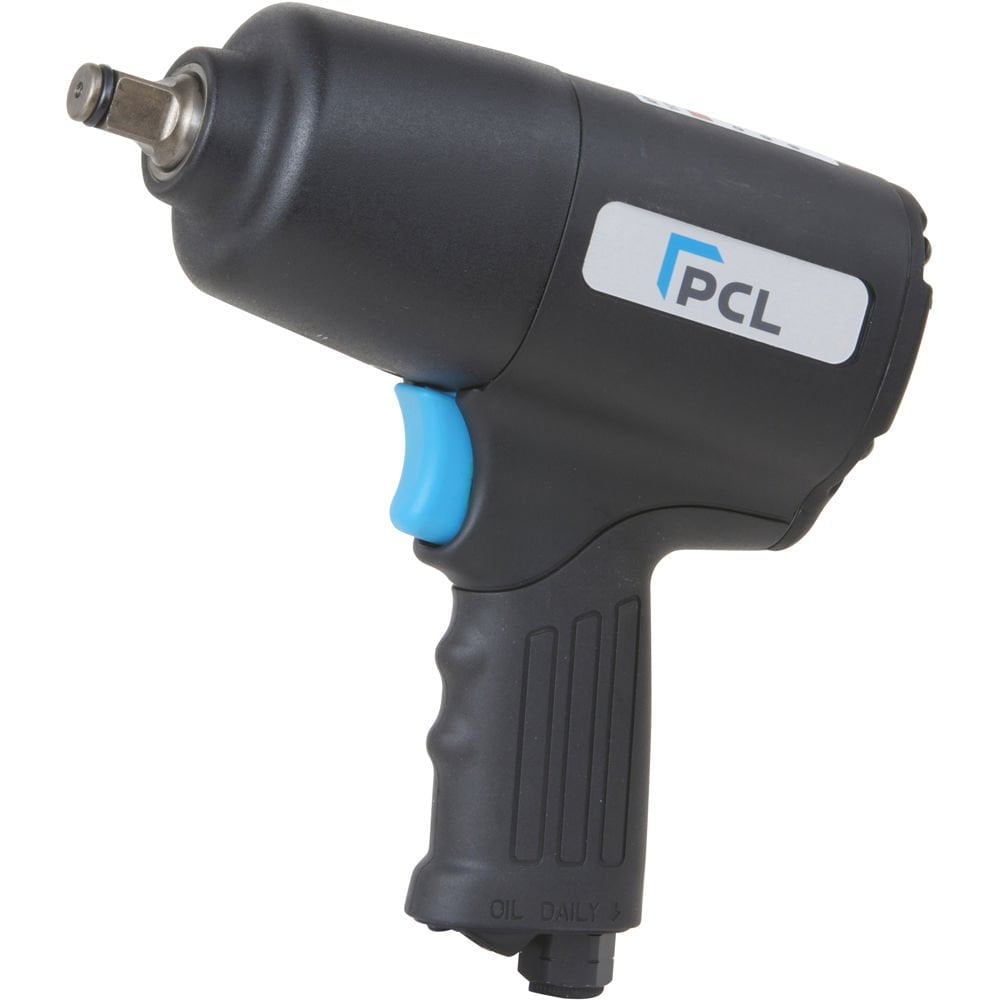
Few examples of such systems interfaced with living cells have been reported in the recent literature: hybrid organic–inorganic films were reviewed by Podsiadlo and colleagues, highlighting the potential of thin structures made of a polymer/clay doped with nanotubes for a series of applications, including biological ones. (29,30) Composite ultrathin films constituted by a polymeric matrix with embedded polymeric or nonpolymeric nanomaterials represent interesting devices, able to combine the unique properties of nanofilms with additional cues provided by the presence of nanofillers.

(28)Īs known, cell behavior is strongly influenced by nanoscale chemical–physical patterns and cues, which constitute an instructive environment able to regulate cell adhesion, orientation and motility, cytoskeletal condensation, and modulation of intracellular signalling pathways that control protein synthesis. (21) Single-layer nanofilms have also been tested with a number of normal and cancer cell types, with the aim of exploiting the peculiar mechanical, (22,23) chemical, (24−26) and topographical (27) nanosheet features in order to influence cell processes or to promote certain stem cell fates. (1,18,19) To this aim, polyelectrolyte multilayer films have been tested as tools for mechanosensitivity studies on mammalian cells, (20) also evaluating the role of gelatin- and fibronectin-based coatings. Single-layer and multilayer nanofilms have been recently proposed as innovative platforms in the fields of regenerative medicine and tissue engineering. Polymeric ultrathin films are particularly suitable to this purpose, thanks to their high tailorability in terms of mechanical properties, surface chemistry, surface electrical charges, and overall matrix flexibility.

One of the most intriguing biomedical applications of nanofilms concerns their close interaction with living cells and their capability to influence key cell processes, such as adhesion, proliferation, and differentiation. (16) Recently, Kam and colleagues highlighted the importance of nanocoatings provided with specific topographies to properly modulate protein adsorption at the surface of the implant and the expression of factors associated with body fibrotic response.
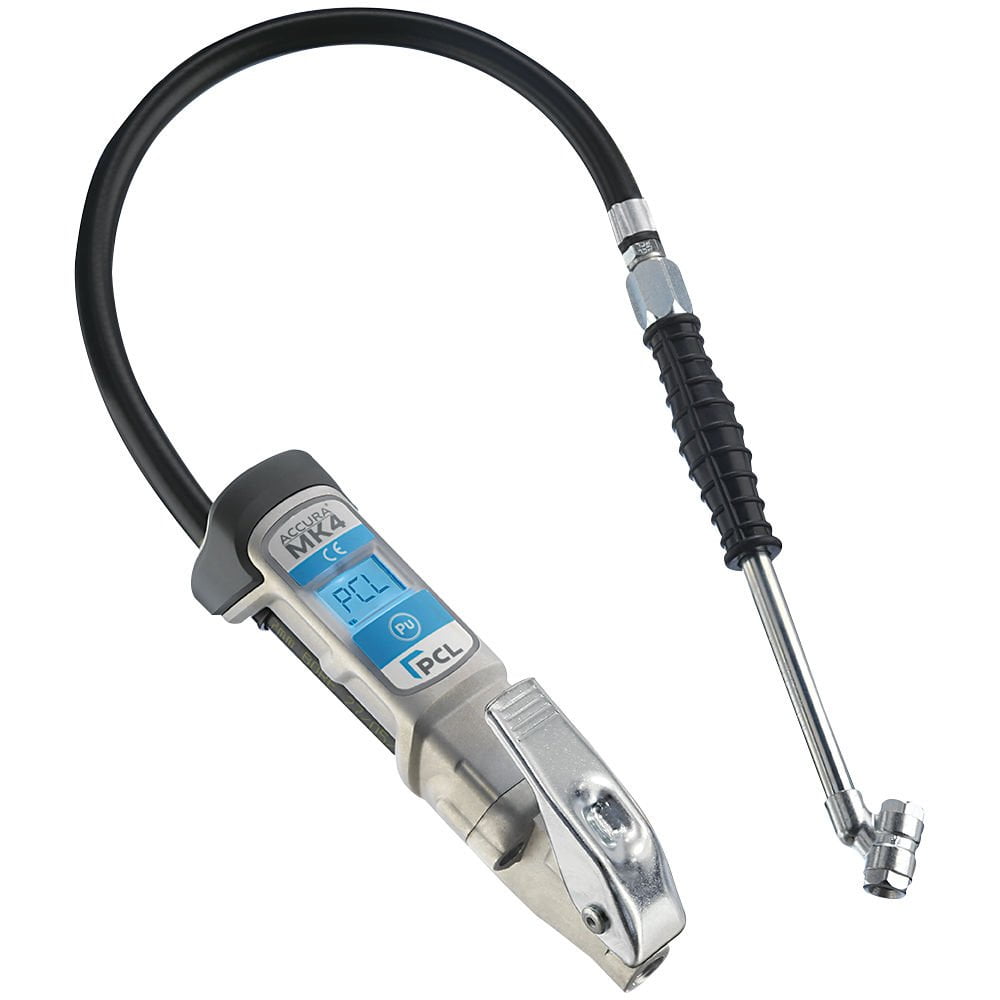
demonstrated that degradable nano-thick coatings enhanced the bonding strength with nanocrystalline hydroxyapatite, with promising applications in bone regeneration. Such elements can be released through simple diffusion mechanisms or by exploiting triggering effects, (12−14) Another widely employed medical application concerns implant surface coating: van den Beucken and colleagues reported the efficacy of DNA-based coatings on titanium, obtained via an electrostatic self-assembly technique. (9) Polymeric nanofilms have also been widely used for developing smart drug delivery systems: (10,11) small drugs, proteins, or nucleic acids can be efficiently embedded in ultrathin matrices by adsorption, chemical binding, or physical confinement. Takeoka and colleagues formerly exploited the large contact area of biodegradable nanosheets to develop nanoadhesive plasters showing several advantages for surgical dressing. These findings demonstrated the potential of these nanocomposite matrices for regenerative medicine applications, such as tissue engineering. Interestingly, a higher content of ZnO NPs in the matrix demonstrated higher bioactivity, boosting the metabolic activity of fibroblasts, myoblasts, and chondrocytes and enhancing the osteogenic and myogenic differentiation. All cell types showed good adhesion and viability on all substrate formulations. In vitro tests were carried out with cells of the musculoskeletal apparatus (fibroblasts, osteoblasts, chondrocytes, and myoblasts). The ZnO NP content was also directly proportional to the material melting point and crystallinity and inversely proportional to the material degradation rate, thus highlighting the stabilization role of ZnO particles. Increasing ZnO NP concentrations implied larger roughness values (∼22 nm for the bare nanofilm and ∼67 nm for the films with 10 mg/mL of NPs), larger piezoelectricity (average d 33 coefficient for the film up to ∼1.98 pm/V), and elastic modulus: the nanofilms doped with 1 and 10 mg/mL of NPs were much stiffer than the nondoped controls and nanofilms doped with 0.1 mg/mL of NPs. All nanofilm types were featured by a thickness value of ∼500 nm. In this paper, a novel nanofilm type is proposed based on a blend of poly(ethylene glycol)- block-poly(ε-caprolactone) methyl ether (PEG- b-PCL) and poly( l-lactic acid), doped with zinc oxide nanoparticles (ZnO NPs) at different concentrations (0.1, 1, and 10 mg/mL).
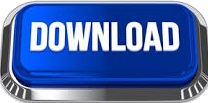